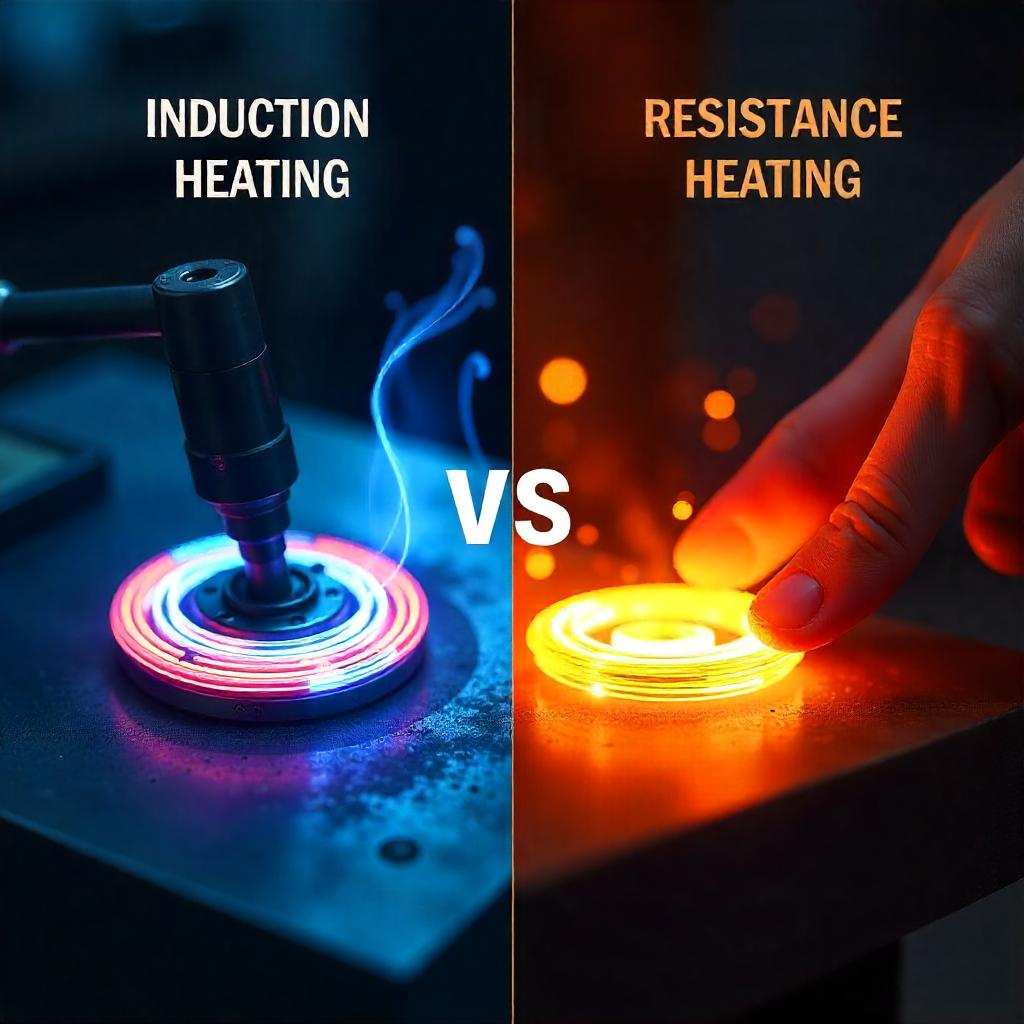
Have you ever wondered how electricity is generated in power plants or how transformers work? The answer lies in a fundamental physical phenomenon called electromagnetic induction. Despite powering our modern world, many people find this concept complex and difficult to understand. Without a clear understanding of electromagnetic induction, grasping how electricity generation, motors, and many everyday technologies function becomes nearly impossible. In this comprehensive guide, we’ll break down how electromagnetic induction works, its principles, and its critical applications in today’s technology.
Electromagnetic induction is the process of generating electrical current in a conductor by placing it in a changing magnetic field. This phenomenon forms the foundation of modern electrical power generation and is responsible for converting mechanical energy into electrical energy in power plants worldwide.
When a conductor moves through a magnetic field or when a magnetic field changes around a stationary conductor, an electromotive force (EMF) is induced. If the conductor is part of a complete circuit, this EMF causes electrical current to flow.
Key concept: Electromagnetic induction requires relative motion or change between a conductor and a magnetic field to generate electricity.
The discovery of electromagnetic induction represents one of the most significant breakthroughs in the history of physics and electrical engineering. In 1831, British scientist Michael Faraday conducted a series of experiments that demonstrated how a changing magnetic field could produce an electric current.
Faraday’s most famous experiment involved wrapping two coils of wire around opposite sides of an iron ring. When he connected one coil to a battery, he observed that a current momentarily flowed in the second coil—but only when the battery circuit was being connected or disconnected. This momentary current was induced by the changing magnetic field created in the iron ring.
Around the same time, American scientist Joseph Henry independently discovered the principle of induction, though Faraday published his findings first and is generally credited with the discovery.
This discovery established the crucial link between electricity and magnetism, leading to the development of generators, transformers, and countless other electrical devices.
To understand electromagnetic induction, we need to grasp several fundamental principles:
The fundamental principle is clear: a changing magnetic field produces an electric field, and if a conductor is present in that electric field, current will flow.
Faraday’s Law of Electromagnetic Induction quantifies the relationship between the changing magnetic field and the induced electromotive force (EMF). This law is represented mathematically and forms the basis for understanding how electromagnetic induction works.
Faraday’s Law states: The induced electromotive force in a circuit is equal to the negative rate of change of magnetic flux through the circuit.
Mathematically, it’s expressed as:
ε = -dΦ/dt
Where:
For a coil with multiple turns, the formula becomes:
ε = -N × dΦ/dt
Where N is the number of turns in the coil.
Key insight: The faster the magnetic flux changes, the greater the induced EMF. This explains why rapid movement of a magnet near a coil produces a stronger current than slow movement.
Lenz’s Law, formulated by Heinrich Lenz in 1834, complements Faraday’s Law by explaining the direction of the induced current. This principle is crucial for understanding the opposing nature of electromagnetic induction.Lenz’s Law states: The direction of the induced current is such that it creates a magnetic field that opposes the change in magnetic flux that produced it.In simpler terms, nature resists changes. When a changing magnetic field induces a current, that current creates its own magnetic field that works against the original change.This explains the negative sign in Faraday’s Law equation and has important implications:
Practical example: When you push a magnet into a coil, the induced current creates a magnetic field that repels the magnet, making you feel resistance as you push.
The process of electromagnetic induction can be visualized at the atomic level. When a conductor enters a magnetic field, the free electrons within the conductor experience a force due to the magnetic field. If the conductor is moving (or the magnetic field is changing), these electrons are pushed in a specific direction, creating an electric current.
For effective electromagnetic induction, several components are typically involved:
The strength of the induced current depends on several factors:
Important concept: Maximum induction occurs when the conductor moves perpendicular to the magnetic field. No induction occurs when the conductor moves parallel to the field lines.
Several factors determine the strength and efficiency of electromagnetic induction:
Engineers and designers carefully consider these factors when creating devices that rely on electromagnetic induction, optimizing for efficiency, power output, and specific applications.
Electromagnetic induction is not just a theoretical concept—it’s the foundation of numerous technologies that power our modern world:
These applications demonstrate the versatility and importance of electromagnetic induction in our daily lives.
The most significant application of electromagnetic induction is in electrical power generation. Nearly all electricity generated worldwide—whether from coal, natural gas, nuclear, hydro, or wind—relies on electromagnetic induction.
The basic principle of a generator includes:
As the turbine rotates the conductor coils through the magnetic field, an EMF is induced, generating electricity. The frequency of the generated AC electricity depends on the rotation speed, while the voltage depends on field strength, coil turns, and rotation speed.
Types of power generation using electromagnetic induction include:
Despite the different energy sources, all these methods use electromagnetic induction as the final step to generate electricity.
Beyond power generation, electromagnetic induction powers many devices we use daily:
Induction Motors: Found in refrigerators, air conditioners, fans, and washing machines, these motors use alternating current to create a rotating magnetic field that induces current in the rotor, causing it to spin.
Induction Cooktops: These modern cooking surfaces create a high-frequency alternating magnetic field that induces eddy currents directly in the cookware, generating heat without heating the cooking surface itself.
Wireless Chargers: Using the principle of induction, these devices transfer energy between two coils—one in the charging pad and one in the device—eliminating the need for direct electrical connections.
Electric Toothbrushes: Many rechargeable toothbrushes use induction charging, with a coil in the base and another in the toothbrush handle.
Transformers: These devices, which use mutual induction between coils, are present in countless electronic devices to convert voltage levels, from the large ones on power poles to tiny ones in phone chargers.
Understanding electromagnetic induction helps us appreciate how these everyday technologies function and the principles that make them work efficiently.
Electromagnetic induction continues to evolve, with several emerging technologies and improvements on the horizon:
These advancements continue to expand the applications of electromagnetic induction, making it an ever more critical technology for our future.
Electromagnetic induction—the process of generating electricity from a changing magnetic field—stands as one of the most important discoveries in the history of physics and engineering. From powering our global electrical grid to enabling wireless charging of our smartphones, this phenomenon underlies countless technologies we rely on daily.
As we’ve explored, electromagnetic induction follows precise physical laws articulated by Faraday and Lenz, which explain both the magnitude and direction of induced currents. By understanding these principles, engineers have developed increasingly efficient and innovative applications.
As technology advances, electromagnetic induction will continue to play a crucial role in solving energy challenges, improving device efficiency, and enabling new wireless technologies. Whether you’re interested in engineering, physics, or simply understanding how the world works, electromagnetic induction remains an essential concept worth understanding. To know more visit our website.
Electromagnetic induction is the production of an electromotive force (EMF) across an electrical conductor when it is exposed to a changing magnetic field. This process converts magnetic energy into electrical energy.
Michael Faraday discovered electromagnetic induction in 1831, with Joseph Henry making similar discoveries independently around the same time.
The primary laws are Faraday’s Law, which states that the induced EMF is proportional to the rate of change of magnetic flux, and Lenz’s Law, which determines the direction of the induced current.
Electromagnetic waves are not directly induced by electromagnetic induction. They are created by oscillating electric and magnetic fields that propagate through space, while electromagnetic induction specifically refers to generating electric current from changing magnetic fields.
Yes, electromagnetic induction can occur without physical movement if the magnetic field itself changes in strength or direction. For example, a transformer works by using an alternating current to create a changing magnetic field in a stationary system.
Good electrical conductors like copper and aluminum work best for the conductive elements, while ferromagnetic materials like iron, steel, and ferrite are optimal for concentrating and directing magnetic fields.
Electromagnetic induction involves generating current from changing magnetic fields, while electrostatic induction involves the redistribution of electric charges in an object due to the influence of a nearby charged body.
Yes, permanent magnets can be used for electromagnetic induction if there is relative motion between the magnet and the conductor. However, electromagnets are often preferred in applications like generators because their field strength can be controlled.
Higher frequencies of magnetic field changes result in greater induced EMF, as per Faraday’s Law. This is why AC generators typically operate at specific frequencies (50 or 60 Hz) for optimal performance.
Modern large-scale generators can achieve efficiencies of over 90% in converting mechanical energy to electrical energy through electromagnetic induction, though the overall efficiency of power plants is lower due to other factors in the energy conversion chain.